@WFS,World Fossil Society,Riffin T Sajeev,Russel T Sajeev
Reservoirs of oxygen-rich iron between Earth’s core and mantle could have played a major role in Earth’s history, including the breakup of supercontinents, drastic changes in Earth’s atmospheric makeup, and the creation of life, according to recent work from an international research team published in National Science Review.
The team — which includes scientists from Carnegie, Stanford University, the Center for High Pressure Science and Technology Advanced Research in China, and the University of Chicago — probed the chemistry of iron and water under the extreme temperatures and pressures of Earth’s core-mantle boundary.
![XRD pattern of reaction products of iron and water. Iron powder was compressed in H2O to 96 GPa, heated up to 2200 K for 5 minutes, and quenched to 300 K. The pattern was composed of the Py-phase (a = 4.370(3) Å), the quenchable high-temperature f.c.c. phase [44] of FeH (a = 3.397(4) Å) and excess ice VII. Inset figure is the caked diffraction pattern, showing the coexistence of the Py-phase (dotted reflections) and FeH (continuous reflections).](http://www.worldfossilsociety.org/wp-content/uploads/2017/11/151209183454_1_540x360-14-1024x474.jpg)
XRD pattern of reaction products of iron and water. Iron powder was compressed in H2O to 96 GPa, heated up to 2200 K for 5 minutes, and quenched to 300 K. The pattern was composed of the Py-phase (a = 4.370(3) Å), the quenchable high-temperature f.c.c. phase [44] of FeH (a = 3.397(4) Å) and excess ice VII. Inset figure is the caked diffraction pattern, showing the coexistence of the Py-phase (dotted reflections) and FeH (continuous reflections).
“Based on our knowledge of the chemical makeup of the slabs that are drawn into Earth’s deep interior by plate tectonics, we think 300 million tons of water could be carried down to meet iron in the core and generate massive iron dioxide rocks each year,” said lead author Ho-kwang “Dave” Mao.
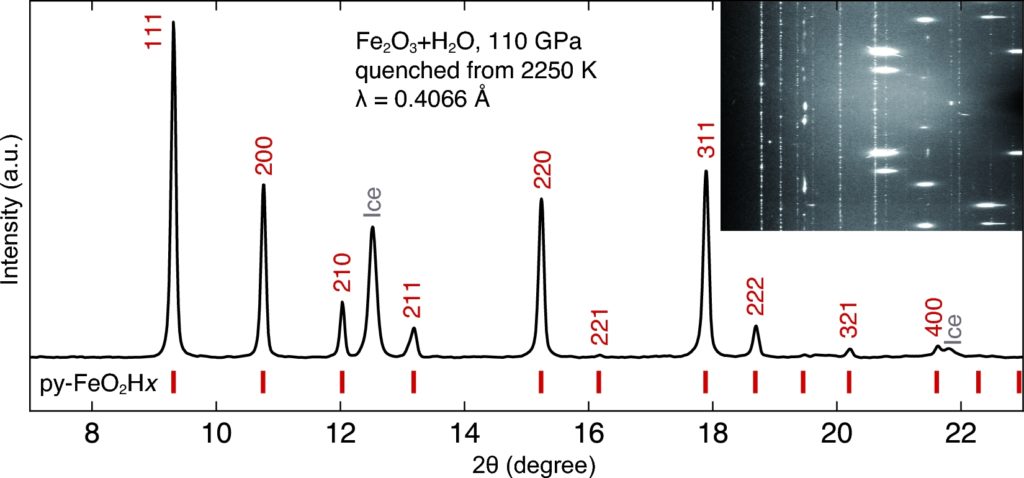
XRD pattern of reaction product of Fe2O3 and water. The sample was compressed to 110 GPa, heated to 2250 K and quenched to 300 K. py, pyrite structured FeO2Hx. Inset figure is the caked image with dotted Py-phase reflections, scattered ice spots and bright diamond spots.
These extremely oxygen-rich solid rocks may accumulate steadily year-by-year above the core, growing into gigantic, continent-like sizes. A geological event that heated up these iron dioxide rocks could cause a massive eruption, suddenly releasing a great deal of oxygen to the surface.
The authors hypothesize that such an oxygen explosion could put a tremendous amount of the gas into Earth’s atmosphere — enough to cause the so-called Great Oxygenation Event, which occurred about 2.5 billion years ago and created our oxygen-rich atmosphere, conditions that kickstarted the rise oxygen-dependent life as we know it.
“This newly discovered high-temperature and intense-pressure water-splitting reaction affects geochemistry from the deep interior to the atmosphere” said Mao. “Many previous theories need to be re-examined now.”
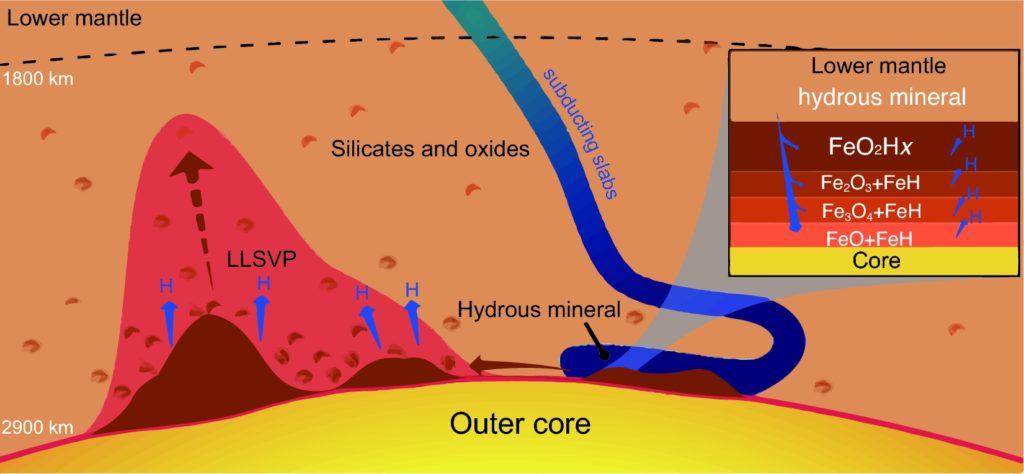
Schematic diagram of ORP in the DLM. Hydrous minerals in the subducting slab (blue) carry H2O to react with the iron core to form the ORP (dark brown) which is a multilayer with increasing oxygen content (inset). H2O penetrates the multilayer to produce more Py-phase, and hydrogen escapes from FeH and FeO2Hx and ascends upwards to sustain the hydrogen cycle. The ORP moves laterally and accumulates. Some ORP (small patches) are scattered and mixed with the DLM silicates and oxides.
- Ho-Kwang Mao, Qingyang Hu, Liuxiang Yang, Jin Liu, Duck Young Kim, Yue Meng, Li Zhang, Vitali B. Prakapenka, Wenge Yang, Wendy L. Mao. When water meets iron at Earth’s core–mantle boundary. National Science Review, 2017; DOI: 10.1093/nsr/nwx109