@WFS,World Fossil Society,Riffin T Sajeev,Russel T Sajeev
Researchers from North Carolina State University, Lund University in Sweden and the University of Hyogo in Japan have retrieved original pigment, beta-keratin and muscle proteins from a 54 million-year-old sea turtle hatchling. The work adds to the growing body of evidence supporting persistence of original molecules over millions of years and also provides direct evidence that a pigment-based survival trait common to modern sea turtles evolved at least 54 million years ago.

Holotype of Tasbacka danica. (a) Photograph of the fossil. Fo, fontanelle (the light colour is a result of sediment infill); Hyo, hyoplastron; Hyp, hypoplastron; Ne, neural; Nu, nuchal; Pe, peripheral; Py, pygal. Arrowheads indicate neural nodes. (b) Detail of the carapace with the sampled area demarcated by a circle. Co, costal; Hu, humerus; Sc, scapula. (c) Higher magnification image showing marginal scutes (arrowheads), pigmentations on bones (arrows), and a brown-black film covering the fontanelles (stars).
Tasbacka danica is a species of sea turtle that lived during the Eocene period, between 56 and 34 million years ago. In 2008 an extremely well-preserved T. danica hatchling was recovered from the Für formation in Jutland, Denmark. The specimen was less than 3 inches (74 millimeters) long. In 2013 paleontologist Johan Lindgren of Lund University uncovered soft tissue residues from an area located near the sea turtle’s left “shoulder.” He collected five small samples for biomolecular analysis.
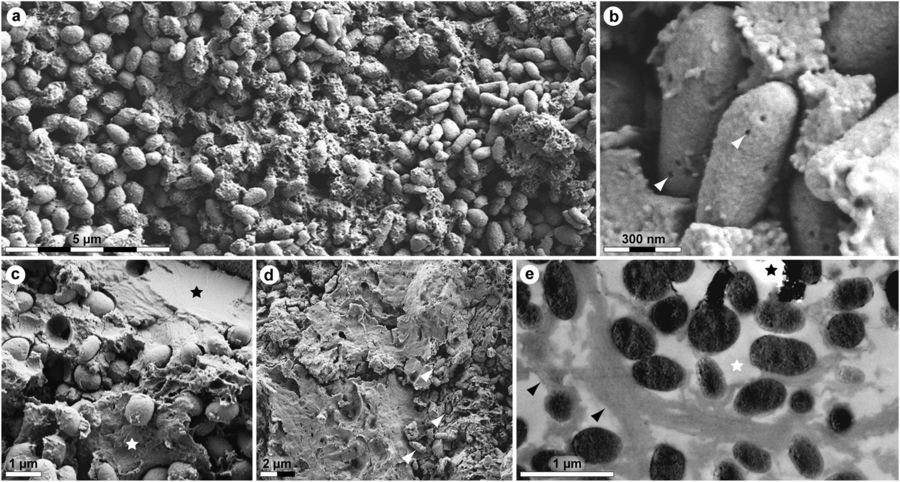
Ultrastructure of MHM-K2 soft tissues. (a) FEG-SEM micrograph of demineralised tissue showing microbodies and adhering matrix. (b) At higher magnification, the microbodies possess a rough surface texture and scattered pits (arrowheads). (c) FEG-SEM micrograph of untreated soft tissue depicting microbodies embedded in a mineral precipitate (black star) and sheet-like matter (white star). (d) Microbodies (arrowheads) in a sheet-like substrate. (e) TEM micrograph of electron-dense microbodies and fibrous matrix (black arrowheads) after demineralisation. White star indicates epoxy resin, whereas black star marks an artificial rupture.
The shells of modern sea turtle hatchlings are dark colored — this pigmentation gives them protection from aerial predators (such as seagulls) as they float on the ocean surface to breathe. Since turtles are reptiles, and therefore cold-blooded, the dark coloration also allows them to absorb heat from sunlight and regulate their body temperature. This elevated body temperature also allows more rapid growth, reducing the time they are vulnerable at the ocean surface.
The T. danica hatchling specimen appeared to share this coloration with its living counterparts. The researchers observed round organelles in the fossil that could be melanosomes, pigment-containing structures in the skin (or epidermis) that give turtle shells their dark color.

Immunoreactivity of fossil and extant turtle tissues. Immunohistochemical staining results for (a,b,e,f,i,j,m,n,q,r; columns 1 and 2) MHM-K2 and Chelonia mydas (c,d; columns 3 and 4) carapace scute and (g,h,k,l,o,p,s,t; columns 3 and 4) muscle tissue to antibodies raised against (a–d; row 1) Gallus gallus domesticus feathers (anti-Gallus fth), (e–h; row 2) Alligator mississippiensis haemoglobin (anti-Alligator Hb), (i–l; row 3) Struthio camelus haemoglobin (anti-Struthio Hb), (m–p; row 4) G. g. domesticus tropomyosin (anti-Gallus trop), and (q–t; row 5) bacterial peptidoglycan (anti-bac pep). a,c,e,g,i,k,m,o,q,s are overlay images, superimposing fluorescent signal on transmitted light image of sectioned tissue to reveal the localisation of antibody-antigen complexes to tissue. b,d,f,h,j,l,n,p,r,t are imaged using a FITC filter. Antibody-antigen complexes are indicated by green fluorescent signal.

Comparison of immunoreactivity between fossil and extant turtle tissues using antibodies raised against chicken feathers conjugated to 12 nm gold beads. (a–d) Low and (e–h) high resolution localisation of gold beads to fibrous matter, but not microbodies/melanosomes, in (a,b,e,f) fossil tissues and (c,d,g,h) modern Chelonia mydas carapace scute material. Insets in b and d demarcate areas depicted in e,f and g,h, respectively. The data support the specificity of the chicken feather antibodies used in this study, and provide independent validation of the immunofluorescent results.
To determine the structural and chemical composition of the soft tissues Lindgren collected and see if the fossil sea turtle did have a dark colored shell, the researchers subjected the sample to a selection of high-resolution analytical techniques, including field emission gun scanning electron microscopy (FEG-SEM), transmission electron microscopy (TEM), in situ immunohistochemistry, time-of-flight secondary ion mass spectrometry (ToF-SIMS), and infrared (IR) microspectroscopy.
Lindgren performed ToF-SIMS on the samples to confirm the presence of heme, eumelanin and proteinaceous molecules — the components of blood, pigment and protein.
Co-author Mary Schweitzer, professor of biological sciences at NC State with a joint appointment at the North Carolina Museum of Natural Sciences, performed histochemical analyses of the sample, finding that it tested positive against antibodies for both alpha and beta-keratin, hemoglobin and tropomyosin, a muscle protein. TEM, performed by University of Hyogo evolutionary biologist Takeo Kuriyama, and Schweitzer’s immunogold testing further confirmed the findings.

Molecular characterisation of MHM-K2 tissues by ToF-SIMS analysis. (a) Positive ion spectrum from a region with strong signal from heme-related ions. (b) Positive ion spectrum of a heme (hemin) standard. (c) Negative ion image showing the signal intensity distribution of ions representing heme (red; 65 + 108 + 134 u), eumelanin (green; 66 + 73 + 74 + 97 + 98 + 121 + 122 u) and silica (blue; 60 + 76 + 77 u). Field of view: 200 × 200 µm2. (d) FEG-SEM micrograph showing a fracture edge. Note abundant microbodies in the crack wall and vesicular texture of the surface. (e) Negative ion spectrum from a region with mixed signal from eumelanin- and heme-related ions. (f) Negative ion spectrum from a region dominated by signal from eumelanin-related ions. (g) Positive ion image showing the signal intensity distribution of ions representing heme (red; 436–488 u), aromatics (blue; 91 + 115 u) and proteinaceous materials (green; 30 + 44 + 70 u). Field of view: 328 × 328 µm2. (h) FEG-SEM micrograph of the demarcated area in g depicting sheet-like matter with high signal from amino acid-related peaks.
In the end, the evidence pointed to these molecules as being original to the specimen, confirming that these ancient turtles shared a pigmentation-based survival trait with their modern-day brethren.
“The presence of eukaryotic melanin within a melanosome embedded in a keratin matrix rules out contamination by microbes, because microbes cannot make eukaryotic melanin or keratin,” Schweitzer says. “So we know that these hatchlings had the dark coloration common to modern sea turtles.
“The data not only support the preservation of multiple proteins, but also suggest that coloration was used for physiology as far back as the Eocene, in the same manner as it is today.”
Sources: 1. Johan Lindgren, Takeo Kuriyama, Henrik Madsen, Peter Sjövall, Wenxia Zheng, Per Uvdal, Anders Engdahl, Alison E. Moyer, Johan A. Gren, Naoki Kamezaki, Shintaro Ueno, Mary H. Schweitzer. Biochemistry and adaptive colouration of an exceptionally preserved juvenile fossil sea turtle. Scientific Reports, 2017; 7 (1) DOI: 10.1038/s41598-017-13187-5
2. North Carolina State University. “Keratin, proteins from 54-million-year-old sea turtle show survival trait evolution.” ScienceDaily. ScienceDaily, 17 October 2017. <www.sciencedaily.com/releases/2017/10/171017091857.htm>
@WFS,World Fossil Society,Riffin T Sajeev,Russel T Sajeev